Manufacturing Processes of the Latest Parallel Plate Capacitors
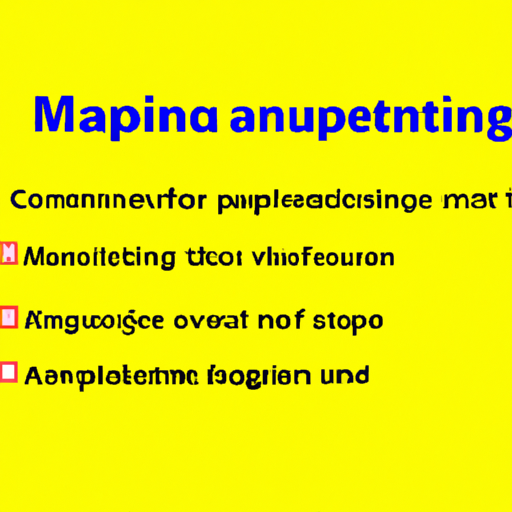
I. Introduction
Parallel plate capacitors are fundamental components in modern electronic devices, playing a crucial role in energy storage and signal processing. These capacitors consist of two conductive plates separated by a dielectric material, which allows them to store electrical energy. As technology advances, the demand for more efficient, compact, and reliable capacitors has led to innovations in their manufacturing processes. This blog post will explore the latest manufacturing techniques for parallel plate capacitors, focusing on materials, design considerations, advanced manufacturing methods, and future trends.
II. Materials Used in Parallel Plate Capacitors
A. Dielectric Materials
The dielectric material is a critical component of parallel plate capacitors, as it determines the capacitor's ability to store charge. Various types of dielectric materials are used, including ceramics, polymers, and composite materials.
1. **Types of Dielectrics**:
- **Ceramic Dielectrics**: Known for their high dielectric constants and stability, ceramic materials are widely used in capacitors for high-frequency applications. They offer excellent temperature stability and low losses.
- **Polymer Dielectrics**: These materials are lightweight and flexible, making them suitable for applications where space and weight are critical. They typically have lower dielectric constants than ceramics but can be engineered for specific applications.
2. **Properties and Selection Criteria**:
When selecting dielectric materials, factors such as dielectric constant, breakdown voltage, temperature stability, and loss tangent are considered. The choice of dielectric directly impacts the capacitor's performance, including its capacitance value and reliability.
B. Conductive Materials
The conductive plates of a capacitor are typically made from metals that exhibit high conductivity and reliability.
1. **Metals Used**:
- **Aluminum**: Commonly used due to its excellent conductivity, lightweight, and cost-effectiveness. Aluminum capacitors are prevalent in consumer electronics.
- **Copper**: Offers superior conductivity and is often used in high-performance applications, although it is more expensive than aluminum.
2. **Conductivity and Reliability Considerations**:
The choice of conductive material affects the capacitor's overall performance, including its equivalent series resistance (ESR) and thermal stability. Manufacturers must balance cost, performance, and reliability when selecting materials.
III. Design Considerations
A. Geometric Configuration
The design of parallel plate capacitors involves careful consideration of geometric parameters.
1. **Plate Area and Separation Distance**:
The capacitance of a parallel plate capacitor is directly proportional to the plate area and inversely proportional to the separation distance. Larger plates and smaller gaps result in higher capacitance values.
2. **Influence on Capacitance**:
Designers must optimize these parameters to achieve the desired capacitance while maintaining the physical constraints of the application.
B. Tolerance and Precision Requirements
High precision in manufacturing is essential to ensure that capacitors meet their specified performance criteria. Tolerances in plate separation and area can significantly affect capacitance and reliability.
C. Thermal and Electrical Performance
Capacitors must be designed to operate effectively under varying thermal and electrical conditions. This includes considerations for temperature coefficients and voltage ratings to ensure long-term reliability.
IV. Manufacturing Processes
A. Substrate Preparation
The manufacturing process begins with substrate preparation, which is crucial for ensuring the quality of the dielectric layer.
1. **Cleaning and Surface Treatment**:
Substrates must be thoroughly cleaned to remove contaminants that could affect adhesion and performance. Techniques such as ultrasonic cleaning and plasma treatment are commonly used.
2. **Substrate Material Selection**:
The choice of substrate material, such as glass, silicon, or ceramics, depends on the application and desired properties of the capacitor.
B. Dielectric Layer Deposition
The dielectric layer is deposited onto the substrate using various techniques.
1. **Techniques**:
- **Physical Vapor Deposition (PVD)**: This method involves the physical transfer of material from a source to the substrate, resulting in a thin, uniform dielectric layer.
- **Chemical Vapor Deposition (CVD)**: CVD involves chemical reactions that deposit the dielectric material onto the substrate, allowing for precise control over thickness and composition.
2. **Thickness Control and Uniformity**:
Achieving uniform thickness is critical for consistent capacitor performance. Advanced monitoring systems are employed to ensure that the dielectric layer meets specified thickness requirements.
C. Electrode Fabrication
The next step involves fabricating the conductive electrodes.
1. **Methods**:
- **Sputtering**: A physical vapor deposition technique where atoms are ejected from a target material and deposited onto the substrate to form the electrode.
- **Electroplating**: A process that uses electrical current to reduce metal cations from a solution onto the substrate, forming a conductive layer.
2. **Patterning Techniques**:
- **Photolithography**: This technique uses light to transfer a pattern onto the dielectric layer, allowing for precise electrode shapes.
- **Laser Etching**: A method that uses focused laser beams to etch patterns onto the substrate, providing high precision and flexibility in design.
D. Assembly and Packaging
Once the electrodes are fabricated, the capacitor is assembled and packaged.
1. **Layer Stacking and Alignment**:
Proper alignment of the dielectric and electrodes is crucial for optimal performance. Automated systems are often used to ensure precision during assembly.
2. **Encapsulation Techniques**:
Encapsulation protects the capacitor from environmental factors and mechanical stress. Techniques such as potting and conformal coating are commonly employed.
3. **Quality Control Measures**:
Rigorous testing and inspection processes are implemented to ensure that each capacitor meets quality standards before it is released to the market.
V. Advanced Manufacturing Techniques
A. Nanotechnology in Capacitor Manufacturing
Nanotechnology is revolutionizing capacitor manufacturing by enabling the use of nanoscale materials.
1. **Benefits of Nanoscale Materials**:
Nanoscale dielectrics can enhance capacitance and reduce leakage currents, leading to improved performance in smaller packages.
2. **Impact on Performance and Size**:
The integration of nanotechnology allows for the development of capacitors that are not only more efficient but also significantly smaller, making them ideal for compact electronic devices.
B. 3D Printing and Additive Manufacturing
Additive manufacturing techniques, such as 3D printing, are emerging as innovative methods for capacitor production.
1. **Innovations in Design and Production**:
3D printing allows for complex geometries and customized designs that traditional manufacturing methods cannot achieve.
2. **Customization and Rapid Prototyping**:
The ability to quickly produce prototypes enables faster design iterations and customization for specific applications, enhancing the overall development process.
VI. Testing and Quality Assurance
A. Electrical Testing Methods
To ensure reliability and performance, various electrical testing methods are employed.
1. **Capacitance Measurement**:
Accurate measurement of capacitance is essential for verifying that the capacitor meets its specifications.
2. **Insulation Resistance Testing**:
This test assesses the dielectric's ability to prevent current leakage, which is critical for the capacitor's reliability.
B. Reliability Testing
Reliability testing is crucial for assessing the long-term performance of capacitors.
1. **Temperature and Humidity Tests**:
These tests simulate environmental conditions to evaluate how capacitors perform under stress.
2. **Life Cycle Testing**:
Life cycle tests assess the capacitor's performance over extended periods, ensuring that it can withstand the rigors of real-world applications.
VII. Future Trends in Parallel Plate Capacitor Manufacturing
A. Sustainable Manufacturing Practices
As environmental concerns grow, manufacturers are increasingly focusing on sustainable practices, including the use of eco-friendly materials and processes that minimize waste.
B. Integration with Emerging Technologies
The rise of the Internet of Things (IoT) and electric vehicles is driving demand for advanced capacitors that can meet the specific needs of these technologies, such as higher energy density and faster charging capabilities.
C. Research and Development Directions
Ongoing research in materials science and manufacturing techniques will continue to push the boundaries of capacitor performance, leading to innovations that enhance efficiency and reliability.
VIII. Conclusion
In summary, the manufacturing processes of the latest parallel plate capacitors involve a complex interplay of materials, design considerations, and advanced techniques. As technology evolves, the demand for more efficient and reliable capacitors will drive continuous innovation in manufacturing processes. The future of parallel plate capacitors looks promising, with advancements in nanotechnology, additive manufacturing, and sustainable practices paving the way for next-generation electronic components. As we move forward, the importance of these capacitors in powering our increasingly digital world cannot be overstated.
Manufacturing Processes of the Latest Parallel Plate Capacitors
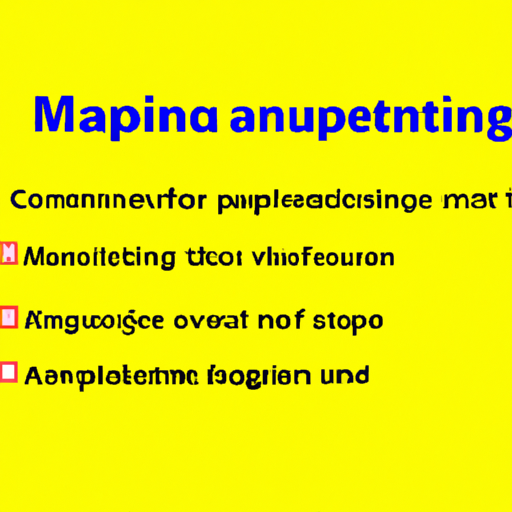
I. Introduction
Parallel plate capacitors are fundamental components in modern electronic devices, playing a crucial role in energy storage and signal processing. These capacitors consist of two conductive plates separated by a dielectric material, which allows them to store electrical energy. As technology advances, the demand for more efficient, compact, and reliable capacitors has led to innovations in their manufacturing processes. This blog post will explore the latest manufacturing techniques for parallel plate capacitors, focusing on materials, design considerations, advanced manufacturing methods, and future trends.
II. Materials Used in Parallel Plate Capacitors
A. Dielectric Materials
The dielectric material is a critical component of parallel plate capacitors, as it determines the capacitor's ability to store charge. Various types of dielectric materials are used, including ceramics, polymers, and composite materials.
1. **Types of Dielectrics**:
- **Ceramic Dielectrics**: Known for their high dielectric constants and stability, ceramic materials are widely used in capacitors for high-frequency applications. They offer excellent temperature stability and low losses.
- **Polymer Dielectrics**: These materials are lightweight and flexible, making them suitable for applications where space and weight are critical. They typically have lower dielectric constants than ceramics but can be engineered for specific applications.
2. **Properties and Selection Criteria**:
When selecting dielectric materials, factors such as dielectric constant, breakdown voltage, temperature stability, and loss tangent are considered. The choice of dielectric directly impacts the capacitor's performance, including its capacitance value and reliability.
B. Conductive Materials
The conductive plates of a capacitor are typically made from metals that exhibit high conductivity and reliability.
1. **Metals Used**:
- **Aluminum**: Commonly used due to its excellent conductivity, lightweight, and cost-effectiveness. Aluminum capacitors are prevalent in consumer electronics.
- **Copper**: Offers superior conductivity and is often used in high-performance applications, although it is more expensive than aluminum.
2. **Conductivity and Reliability Considerations**:
The choice of conductive material affects the capacitor's overall performance, including its equivalent series resistance (ESR) and thermal stability. Manufacturers must balance cost, performance, and reliability when selecting materials.
III. Design Considerations
A. Geometric Configuration
The design of parallel plate capacitors involves careful consideration of geometric parameters.
1. **Plate Area and Separation Distance**:
The capacitance of a parallel plate capacitor is directly proportional to the plate area and inversely proportional to the separation distance. Larger plates and smaller gaps result in higher capacitance values.
2. **Influence on Capacitance**:
Designers must optimize these parameters to achieve the desired capacitance while maintaining the physical constraints of the application.
B. Tolerance and Precision Requirements
High precision in manufacturing is essential to ensure that capacitors meet their specified performance criteria. Tolerances in plate separation and area can significantly affect capacitance and reliability.
C. Thermal and Electrical Performance
Capacitors must be designed to operate effectively under varying thermal and electrical conditions. This includes considerations for temperature coefficients and voltage ratings to ensure long-term reliability.
IV. Manufacturing Processes
A. Substrate Preparation
The manufacturing process begins with substrate preparation, which is crucial for ensuring the quality of the dielectric layer.
1. **Cleaning and Surface Treatment**:
Substrates must be thoroughly cleaned to remove contaminants that could affect adhesion and performance. Techniques such as ultrasonic cleaning and plasma treatment are commonly used.
2. **Substrate Material Selection**:
The choice of substrate material, such as glass, silicon, or ceramics, depends on the application and desired properties of the capacitor.
B. Dielectric Layer Deposition
The dielectric layer is deposited onto the substrate using various techniques.
1. **Techniques**:
- **Physical Vapor Deposition (PVD)**: This method involves the physical transfer of material from a source to the substrate, resulting in a thin, uniform dielectric layer.
- **Chemical Vapor Deposition (CVD)**: CVD involves chemical reactions that deposit the dielectric material onto the substrate, allowing for precise control over thickness and composition.
2. **Thickness Control and Uniformity**:
Achieving uniform thickness is critical for consistent capacitor performance. Advanced monitoring systems are employed to ensure that the dielectric layer meets specified thickness requirements.
C. Electrode Fabrication
The next step involves fabricating the conductive electrodes.
1. **Methods**:
- **Sputtering**: A physical vapor deposition technique where atoms are ejected from a target material and deposited onto the substrate to form the electrode.
- **Electroplating**: A process that uses electrical current to reduce metal cations from a solution onto the substrate, forming a conductive layer.
2. **Patterning Techniques**:
- **Photolithography**: This technique uses light to transfer a pattern onto the dielectric layer, allowing for precise electrode shapes.
- **Laser Etching**: A method that uses focused laser beams to etch patterns onto the substrate, providing high precision and flexibility in design.
D. Assembly and Packaging
Once the electrodes are fabricated, the capacitor is assembled and packaged.
1. **Layer Stacking and Alignment**:
Proper alignment of the dielectric and electrodes is crucial for optimal performance. Automated systems are often used to ensure precision during assembly.
2. **Encapsulation Techniques**:
Encapsulation protects the capacitor from environmental factors and mechanical stress. Techniques such as potting and conformal coating are commonly employed.
3. **Quality Control Measures**:
Rigorous testing and inspection processes are implemented to ensure that each capacitor meets quality standards before it is released to the market.
V. Advanced Manufacturing Techniques
A. Nanotechnology in Capacitor Manufacturing
Nanotechnology is revolutionizing capacitor manufacturing by enabling the use of nanoscale materials.
1. **Benefits of Nanoscale Materials**:
Nanoscale dielectrics can enhance capacitance and reduce leakage currents, leading to improved performance in smaller packages.
2. **Impact on Performance and Size**:
The integration of nanotechnology allows for the development of capacitors that are not only more efficient but also significantly smaller, making them ideal for compact electronic devices.
B. 3D Printing and Additive Manufacturing
Additive manufacturing techniques, such as 3D printing, are emerging as innovative methods for capacitor production.
1. **Innovations in Design and Production**:
3D printing allows for complex geometries and customized designs that traditional manufacturing methods cannot achieve.
2. **Customization and Rapid Prototyping**:
The ability to quickly produce prototypes enables faster design iterations and customization for specific applications, enhancing the overall development process.
VI. Testing and Quality Assurance
A. Electrical Testing Methods
To ensure reliability and performance, various electrical testing methods are employed.
1. **Capacitance Measurement**:
Accurate measurement of capacitance is essential for verifying that the capacitor meets its specifications.
2. **Insulation Resistance Testing**:
This test assesses the dielectric's ability to prevent current leakage, which is critical for the capacitor's reliability.
B. Reliability Testing
Reliability testing is crucial for assessing the long-term performance of capacitors.
1. **Temperature and Humidity Tests**:
These tests simulate environmental conditions to evaluate how capacitors perform under stress.
2. **Life Cycle Testing**:
Life cycle tests assess the capacitor's performance over extended periods, ensuring that it can withstand the rigors of real-world applications.
VII. Future Trends in Parallel Plate Capacitor Manufacturing
A. Sustainable Manufacturing Practices
As environmental concerns grow, manufacturers are increasingly focusing on sustainable practices, including the use of eco-friendly materials and processes that minimize waste.
B. Integration with Emerging Technologies
The rise of the Internet of Things (IoT) and electric vehicles is driving demand for advanced capacitors that can meet the specific needs of these technologies, such as higher energy density and faster charging capabilities.
C. Research and Development Directions
Ongoing research in materials science and manufacturing techniques will continue to push the boundaries of capacitor performance, leading to innovations that enhance efficiency and reliability.
VIII. Conclusion
In summary, the manufacturing processes of the latest parallel plate capacitors involve a complex interplay of materials, design considerations, and advanced techniques. As technology evolves, the demand for more efficient and reliable capacitors will drive continuous innovation in manufacturing processes. The future of parallel plate capacitors looks promising, with advancements in nanotechnology, additive manufacturing, and sustainable practices paving the way for next-generation electronic components. As we move forward, the importance of these capacitors in powering our increasingly digital world cannot be overstated.